Insight from Convergent Evolution
C4 photosynthesis has evolved independently over 60 times, making it one of the most convergent traits in the biosphere and providing a robust system for scientific discovery.
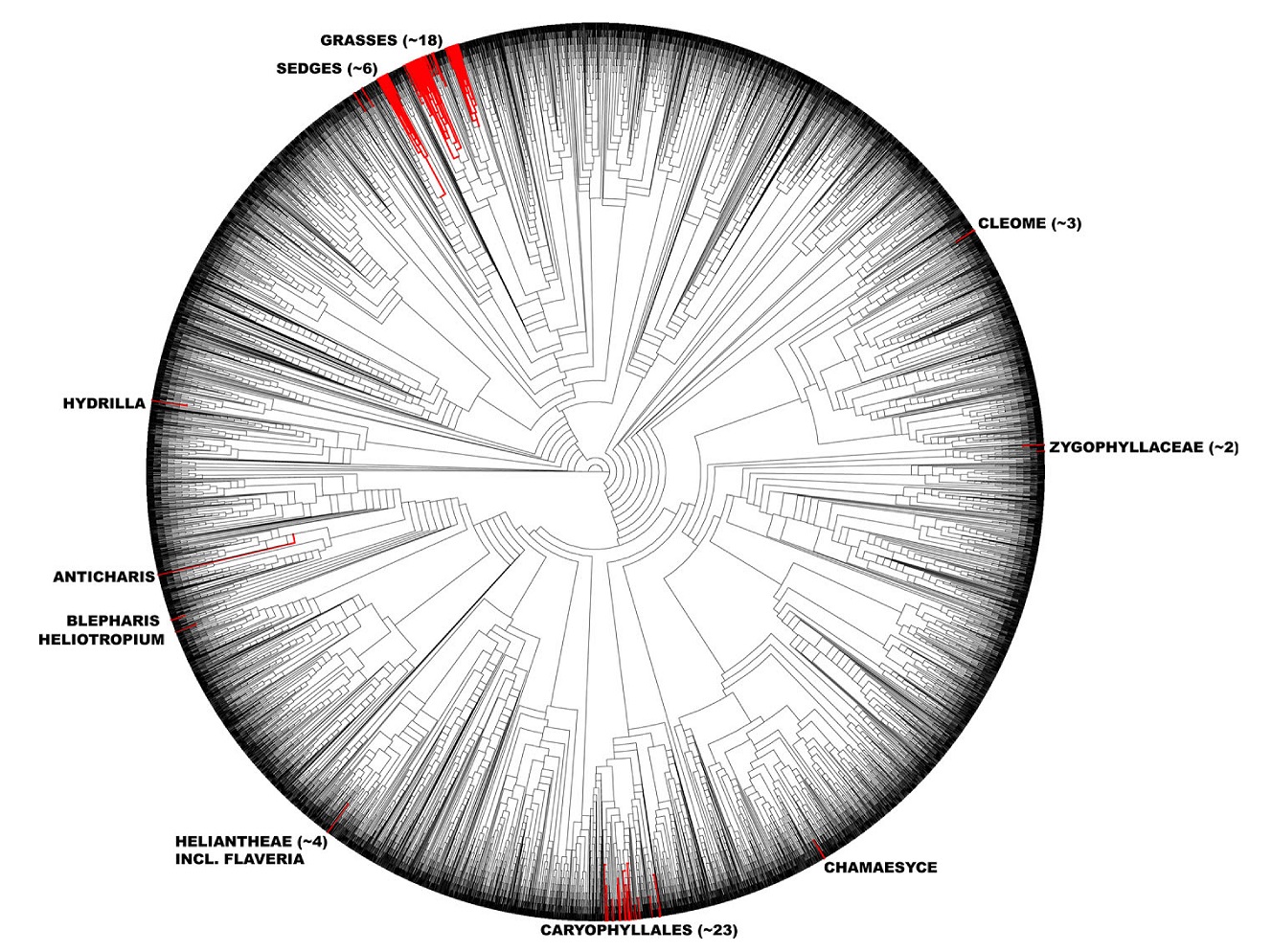
The underlying reason for convergence seems to be that all organisms are under constant scrutiny of natural selection and are also subject to the constraints of the physical and chemical factors that severely limit the action of all inhabitants of the biosphere.
Put simply, convergence shows that in a real world not all things are possible.
-Simon Conway Morris1
The natural world presents numerous striking examples of evolutionary convergence, which occurs when unrelated organisms develop similar solutions to the same problem. For example, the wings of birds and bats are both independently derived from the forelimbs of tetrapods. But convergent evolution is more than just a curious phenomenon. Rather, it represents a powerful means to answer questions about how complex traits evolve, such as: What are the underlying core mechanisms that give rise to a particular trait? Which evolutionary solutions evolve easily, and which are challenging or impossible? What are the constraints on evolution?
With over 60 independent origins2,3 the highly convergent nature of C4 evolution has already provided tremendous insight into how many different ways a complex trait like C4¬ photosynthesis can be assembled. 25 different forms of C4 Kranz anatomy are recognized based on the arrangement of the vascular sheath cells, and two species lack Kranz anatomy altogether, and instead operate a single-cell C4 cycle, with Rubisco compartmentalized in an aggregation of chloroplasts.4 While initial fixation of CO2 by PEP carboxylase is ubiquitous to all C4 plants, three different biochemical subtypes are recognized based on the enzyme co-opted for release of CO2 in the sheath cells: NADP malic enzyme is the most common, followed by NAD malic enzyme, and PEP carboxykinase. These subtypes differ in more than just how they release CO2 to Rubisco; however, the “choice” of decarboxylase has consequences for intercellular transport, tissue-specific organelle investment, ultrastructure, and energetics.5 Using comparative genomics we can gain new insight into how these myriad divergent underlying mechanisms are produced and assembled by natural selection, as well as what constitutes the core genetic requirements of C4 photosynthesis.
citations:
- Morris, S. C. The Crucible of Creation: The Burgess Shale and the Rise of Animals. (Oxford Paperbacks, 1999).
- Sage, R. F. A portrait of the C4 photosynthetic family on the 50th anniversary of its discovery: species number, evolutionary lineages, and Hall of Fame. J. Exp. Bot. 67, 4039–4056 (2016).
- Sage, R. F., Christin, P.-A. & Edwards, E. J. The C4 plant lineages of planet Earth. J Exp Bot 62, 3155–3169 (2011).
- Edwards, G. E. & Voznesenskaya, E. V. Chapter 4 C4 Photosynthesis: Kranz Forms and Single-Cell C4 in Terrestrial Plants. in C4 Photosynthesis and Related CO2 Concentrating Mechanisms (eds. Raghavendra, A. S. & Sage, R. F.) 29–61 (Springer Netherlands, 2011). doi:10.1007/978-90-481-9407-0_4.
- Drincovich, M. F., Lara, M. V., Andreo, C. S. & Maurino, V. G. Chapter 14 C4 Decarboxylases: Different Solutions for the Same Biochemical Problem, the Provision of CO2 to Rubisco in the Bundle Sheath Cells. in C4 Photosynthesis and Related CO2 Concentrating Mechanisms (eds. Raghavendra, A. S. & Sage, R. F.) 277–300 (Springer Netherlands, 2011). doi:10.1007/978-90-481-9407-0_14.